Translate this page into:
Fetal surgery for spina bifida – An upcoming standard of care
*Corresponding author: Sheena Ali, Department of Pediatric Neurosurgery, Arnold Palmer Hospital for Children, Orlando Health, Orlando, Florida, USA. sheena.ali@orlandohealth.com
-
Received: ,
Accepted: ,
How to cite this article: Ali S, Deopujari C, Andar U, Elbabaa S. Fetal surgery for spina bifida – An upcoming standard of care. Wadia J Women Child Health. 2023;2(3):121-31. doi: 10.25259/WJWCH_38_2023
Abstract
Open spina bifida or myelomeningocele (MMC) is one of the most common serious congenital malformations that have been historically treated with a repair after birth. However, this “repair” does not avert neurological injury, reverse hindbrain herniation, or prevent hydrocephalus. With the intention to reduce numerous medical procedures, lifelong morbidities, and medical complications, fetal surgery was proposed about a quarter century ago. Fetal surgery has become the standard of care for MMC, especially after the advent of the Management of Myelomeningocele Study (MOMS) trial. Dr. Michael Harrison from the University of California was the first to birth this idea in the 1980s. While earlier research was carried out on ovine models, it was deduced that intrauterine repair reduces the secondary damage to exposed neural tissue and improves post-natal neurological outcomes. After the promising results in animal models and some clinical series, it paved the way for a prospective multicenter randomized controlled trial that compared prenatal versus postnatal MMC repair, with the aim to prove its efficacy and safety with Level-1 evidence- the MOMS. However, it was stopped early due to the improved prenatal repair outcomes, thus establishing open fetal MMC repair as the standard of care. We aim to discuss the origin story of this remarkable procedure, its rationales and advantages for the unborn child and mother and its short-term and long-term superiority and limitations. The operative procedure and the criteria with other essential aids in its diagnosis have also been discussed briefly. We also intend to eliminate the apprehension faced toward this procedure by the supporting team of obstetricians, anesthetists, neonatologists, and radiologists essential to making this procedure a success.
Keywords
Open neural tube defect
Myelomeningocele
Myeloschisis
Management of myelomeningocele study
Intrauterine repair
INTRODUCTION
Myelomeningocele (MMC) is a type of neural tube defect (NTD) that occurs as a result of incomplete closure of the posterior neuropore during primary neurulation.[1] NTDs are among the most common congenital malformations in the United States, affecting 0.6/1000 live births or 30 of every 100,000 live births in North America.[2,3]
Its prevalence is higher in many low-resourced developing populations, like parts of subSaharan Africa and Southeast Asia.[4] The prevalence in India has been found to be as high as 13–17 cases/10,000 live and stillbirths.[5-9]
The prevalence of the two dreaded NTD conditions – Anencephaly and spina bifida is 21 and 19 cases/10,000 births, respectively.[7] With India’s alarmingly high birth rate, these numbers construe to several hundred thousand NTD cases, as per estimates from the global burden of disease. Dietary folate supplementation, which is an important substrate for neurulation, remains the most important public health intervention to control the prevalence of open neural tube defects (ONTDs) all over the world.[10]
RATIONALE
The logic of prenatal repair of MMCs began with the “twohit hypothesis,” borne from the observation that neurological sequelae advance during gestation.[2,11] The first and irreversible neurological injury occurs due to the failure of caudal neuropore fusion during primary neurulation failure, the “second hit” occurs when the exposed neural placode is subjected to neurotoxic and cytotoxic intrauterine amniotic contents, that causes additional damage.[11] Other consequences like Arnold–Chiari malformation-II (downward displacement of the medulla, fourth ventricle, and cerebellum into the spinal canal) occur as a resultant caudal suctioning effect of cerebrospinal fluid (CSF) within the spinal defect.
Intrauterine repair is based on the principle that subsequent neurological damage and morbidity can be avoided by providing a protective plane between the neural tissue and the surrounding neurotoxic amniotic environment.[12] Usually, ONTDs are closed within days following birth to help mitigate the risk of meningitis and theoretically, to alleviate further neurological damage to more rostral nerve roots and spinal cord. While the irreversible peripheral nerve and spinal cord damage (despite early postnatal repair) is evident at birth, the long-term survivors suffer disabling morbidities such as lower-limb paralysis, bowel and bladder dysfunction. The severity of the neurologic disability in the lower limbs is directly proportional to the level of spinal cord injury.
Almost all infants who are born with MMCs have an associated Arnold–Chiari II malformation, brain-stem abnormalities, low-lying venous sinuses, and a small posterior fossa. This malformation is also associated with hydrocephalus and developmental brain abnormalities that are often treated with CSF diversion procedures like ventriculoperitoneal shunts, which require lifelong monitoring and are prone to malfunctions, infections, and revisions.[12,13] It also has well-documented effects on motor, cranial-nerve, and cognitive function, which are exacerbated by the adverse effects of hydrocephalus and shunting.[14]
Radiological confirmation on sequential ultrasonographic images obtained from fetuses with MMC advocate the progressive “second hit” insult, in the form of worsening hind brain herniation and hydrocephalus with the loss of lower-limb movement. Several animal studies encourage the prenatal coverage of a spina bifida–like lesion as it preserves neurological function and prevents hindbrain herniation.[12,15-21]
A BRIEF HISTORY
The course of development of this meticulous surgery started in the 1980s as the brainchild of Dr. Michael Harrison from University of California San Francisco (UCSF). He was responsible for performing the first fetal surgery on an ovine model to evaluate the efficacy of in utero treatment of congenital diaphragmatic hernia, urinary tract obstructions, and hydrocephalus. This evolved toward testing the maternal safety of a fetal intervention in the most rigorous model possible, a non-human primate (macaque monkey) model in 1981.[22] Following the success of the first in utero intervention for urinary tract obstruction at University of California San Francisco in 1982, tremendous enthusiasm and popularity prompted an international meeting attempted for lethal diseases to lay down a set of ethical guidelines.[22,23] This meeting was called the International Fetal Medicine and Surgery Society and is conducted annually, even today.
Dr. Michejda was the first to investigate prenatal MMC repair as a non-lethal disease for fetal intervention, wherein he observed that the neurologic damage from MMC appeared to be primarily from the secondary injury and destruction of the exposed neural tissue. He showed that the in utero repair could yield excellent neurological results in a primate model in comparison to untreated monkeys who suffered paraplegia, sensory loss, and incontinence.[15,24,25] This was then also confirmed on human fetuses.[26] Perhaps, the most accurate model reflective of human MMC pathophysiology was the ovine model developed by Meuli et al.[16] The sheep that underwent in utero repair exhibited superior neurological function and restoration of normal hindbrain architecture.[17,18]
Paek et al. and Farmer et al. demonstrated that the hindbrain herniation associated with Arnold–Chiari II malformation could also be reversed with fetal repair.[18] With these translational breakthroughs of the potential benefits of the in utero repair, the first human fetal MMC repair was performed by Dr. Joseph Bruner at Vanderbilt University in 1997. In Joyeux L. et al.’s 2018 article, it states that Dr. Farmer proceeded to propose the Management of Myelomeningocele Study (MOMS) trial, which established prenatal repair as the standard of care.[2] The MOMS trial, published in 2011 in the New England Journal of Medicine, established prenatal repair as the standard of care for select fetuses with MMC. Specific inclusion and exclusion criteria were established.
PRENATAL DIAGNOSIS
Before the 21st century, parents expecting a child with an ONTD had two options: Medical termination of pregnancy or delivery followed by postnatal closure of the MMC. As per the rationale for prenatal surgery, the hope was to prevent the progressive neurologic decline that occurs during the “second hit.” Spina bifida is usually diagnosed by measurement of alpha fetoprotein (AFP) in maternal serum at 16 weeks of gestation. Amniocentesis is also required to rule out associated karyotype abnormalities and assess amniotic fluid alpha-fetoprotein levels (AFAFP).
Imaging studies are an essential tool to understand the anatomy of the lesion.[27-31] Fetal ultrasound at 18–24 weeks is used to evaluate the fetus for direct (bony defect, level of lesion, and sac protrusion) and indirect signs (ventriculomegaly, Chiari malformations, limb movement, and other congenital anomalies) [Table 1, Figure 1a and b]. It is essential to exclude skin-covered lesions, such as myelocystocele, lipomyelomeningocele, and myelocele, as these are excluded from fetal intervention. While the ultrasound (USG) alone is cheaper, allows a clearer determination of bony anatomy, and yields higher diagnostic accuracy, magnetic resonance imaging (MRI) does not have limits of operator dependency, even though it is more time-consuming.[32]
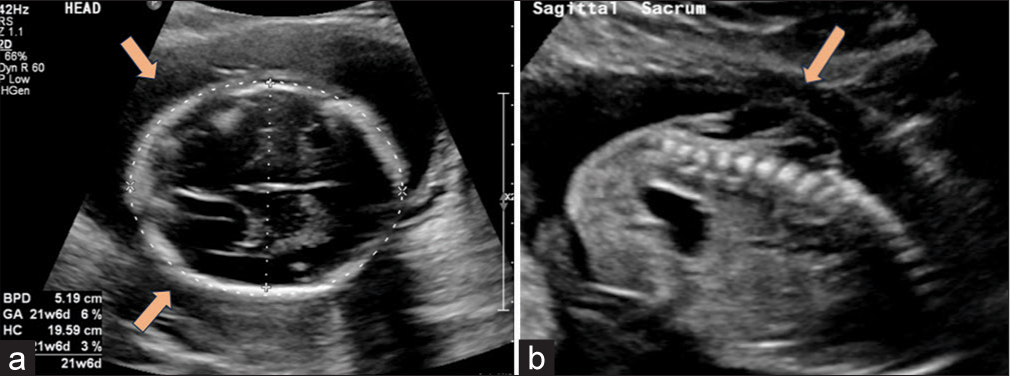
- Ultrasound (USG) findings (a) “Lemon shaped” contour of cranium with clear invagination/scalloping of the frontal bones. (lemon sign) (orange arrows) Near-complete obliteration of Cisterna Magna with cerebellar displacement (banana sign). (b) Dorsal lumbosacral spinal dysraphism with absence of spinous processes and dorsal aspects of vertebrae. Overlying moderately large cystic sac (orange arrow), consistent with spina bifida cystica.
Cranial signs | “Lemon sign” | Concavity of frontal skull bones, postulated to occur due to low intracranial hydrostatic pressure due to persistent CSF leak from neural tube defect. |
(With respect to gestational age) | Small biparietal diameter | |
“Banana sign” | Crescentic shape of cerebellar hemispheres within a small and crowded posterior fossa. | |
Chiari II malformation | Obliteration of cisterna magna | |
“Clivus Supra Occipital angle” below the 10th percentile. (Signifying hypoplasia of cranium overlying the posterior fossa.) | ||
Ventriculomegaly | On axial view, at level of thalami, the atria of the lateral cerebral ventricles measure >10 mm in diameter. | |
“Occipital pointing” – pointed shape of the occipital horns of the lateral ventricles. | ||
Spinal signs | Abruption of “Railroad track” configuration | Widened ossification centers (coronal plane) |
Splayed neural arch ossification centers (transverse plane) | ||
“Shimmering effect” | Cystic meningeal sac | |
Scoliosis/kyphosis | Location, number of segments, and direction of the abnormal spinal curvature can be estimated. | |
Localization of the Conus medullaris and presence of Tethered cord | When the conus medullaris extends far below L3 and to the site of the defect. (Sagittal view) | |
Tethering is usually identified as a “central dot” closer to the lamina in the non-dependent part of the spinal canal and is ideally diagnosed if the conus lies inferior to L3–4 after 18 weeks. | ||
Other | Lower limb | Lower-limb movement and activity at hip, knee, ankles, and toes. |
To determine the presence of anomalies like “Clubbed feet,” “Hammer Toe,” Clinodactyly, etc. |
CSF: Cerebrospinal fluid
Fetal MRI should be performed to confirm and assess the degree of hindbrain herniation and evaluate the detailed fetal brain and spinal cord anatomy [Table 2, Figure 2a and b]. It also provides additional anatomical and physiological information such as tissue microstructure and organ function. Radiological evaluation also helps in operative decision-making as wide defects with no MMC sac (myeloschisis) may be more difficult to close primarily and may require an augmented graft to close the fetal skin.[33,34]
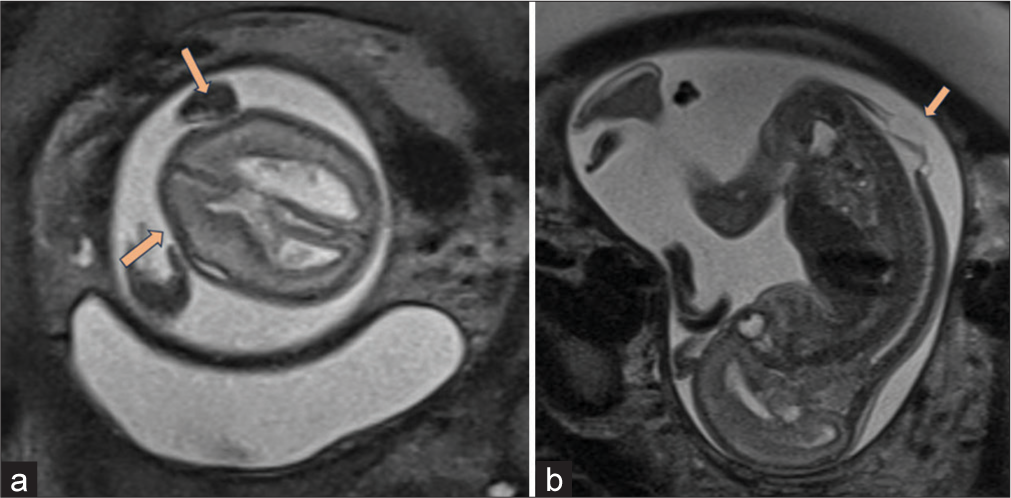
- Magnetic resonance imaging findings (a) moderately dilated lateral ventricles, with scalloping of frontal bones also known as “lemon sign” (orange arrows) with bilateral colpocephaly. (b) Dorsal spinal dysraphism over lumbar area with cystic overlying sac like structure (orange arrow).
Spinal signs | Defect dimensions | Level of Osseous defect. (As high as T10 to as low as L3, to determine the lesion level) |
Size of the bony defect in length (Segment span) and width (Interpediculate distance). | ||
Defect type | Open defect – (Deficient skin and subcutaneous tissue – if the protrusion forms a cystic CSF containing sac, with an overlying thin arachnoid membrane. The cord is usually flattened, without a measurable well-defined sac – “Myeloschisis” defect) | |
Closed defect – (Neural elements which appear as linear, echogenic areas surrounded by anechoic CSF with intact skin coverage). | ||
Kyphoscoliosis | Along with the location and direction of vertebral curvature, the segments involved, and centering of curvature can be determined. | |
Best appreciated in the coronal view. | ||
These descriptions are qualitatively and quantitatively measured. (Mild, moderate, and severe) | ||
Other | Lipoma of spinal cord, dermal sinus tracts, diastematomyelia, Syrinx, hydromyelia, etc. | |
Cranial signs | Chiari malformation | Altered crescentic shape of cerebellum with a small and crowded posterior fossa-“Banana Sign.” |
Effacement or near absence of CSF of the cisterna magna due to small cerebellar size- “Transcerebellar diameter” | ||
Hind brain herniation-brainstem appears tethered down with an elongated low lying the ventricle. | ||
”Tectal Beaking”-posteriorly elongated inferior colliculus. | ||
Frontal bone signs | “Lemon Sign”-concavity of frontal bones postulated due to the low hydrostatic pressure due to leakage of CSF into the amniotic cavity from the neural tube defect. | |
Ventriculomegaly | The atria of lateral ventricles measure more than 10 mm on the axial view at the level of the thalami. | |
Supratentorial anomalies | Sulcal abnormalities such as overdeveloped sulcation and gyration for gestational age, thin cortex, cortical clefts, parenchymal nodules, and germinal matrix hemorrhages. |
CSF: Cerebrospinal fluid, MRI: Magnetic resonance imaging
The presence of hindbrain herniation, absence of a thick-walled sac, and elevated values of amniotic fluid alpha-fetoprotein, and the presence of acetylcholinesterase confirm an open NTD.
PROCEDURE
The inclusion and exclusion criteria set out in the MOMS trial are still employed by most centers to guide which maternal-fetal pairs are candidates for in utero surgery [Table 3]. The mother is usually admitted a day before surgery, wherein the pre-operative laboratory samples are drawn, and an ultrasound examination is done to confirm the defect – size and location and to demonstrate estimated fetal weight and position. The anesthetists and obstetricians then evaluate and give the final clearance for surgery. The fetal surgical team comprises maternal fetal medicine, pediatric neurosurgery, neonatology, pediatric cardiology, plastic surgery, anesthesia, operating room technicians, nurses, and sonographers.
Inclusion criteria |
|
Exclusion criteria |
|
MOMS: Management of myelomeningocele study
A combination of general and epidural anesthesia is administered to the mother, and the uterus is exposed. The fetus and placenta are located through ultrasound and a 6–8 cm hysterotomy incision is created, avoiding the placenta and proximity of the MMC sac, and ensuring that there is no direct trauma to these membranes during the intrauterine portion of the procedure. The margins are sewn or stapled, and amniotic infusion of warm Ringer Lactate solution is initiated.
The fetus is administered an intramuscular fentanyl and vecuronium cocktail while undergoing continuous echocardiographic monitoring throughout the procedure. Under the microscope, the placode is sharply incised, allowing the neural contents to descend within the canal; this is often followed by a few pial sutures to “tubularize” the placode. A synthetic dural substitute placed “onlay” to ensure water-tight closure if sufficient dura is unavailable [Figure 3a-d].
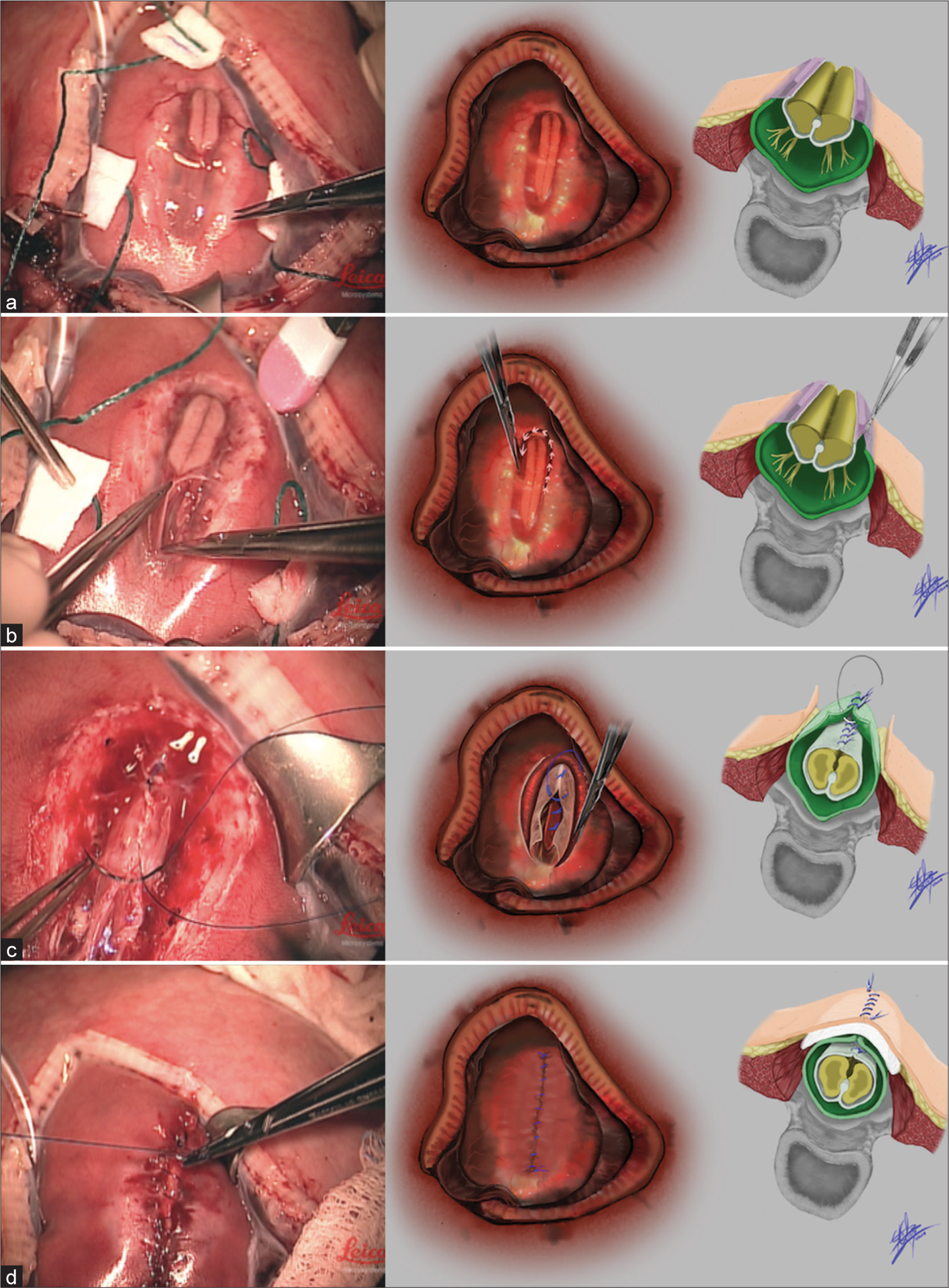
- Steps of surgery (a) from left to right, an intraoperative photograph of the exposed fetal myelomeningocele (MMC) defect before beginning repair, an artist’s drawing of the same stage of the operation, and an anatomical representation of the fetus’ MMC defect before repair. (b) From left to right, an intraoperative photo of MMC placode dissection, an artist’s rendition of the same stage, and an anatomical depiction of the placode dissection. (c) From left to right, an intraoperative photo of primary dural closure during fetal MMC repair, an artist’s rendition of this same step, and an anatomical drawing of primary dural closure also depicting underlying neurulation of the spinal cord. (d) From left to right, intraoperative photo of primary skin repair, artist’s depiction of the same stage, and anatomical drawing of the repaired layers including demonstration of the dermal graft in lay used beneath the skin.
If inadequate, the skin is mobilized and a premature decision is made for a relaxing flank incision to create a bipedicle skin flap, allowing a tensionless primary closure over the defect. An acellular dermal allograft can also be sewn in an “inlay” or “onlay” fashion over larger defects or even over the flank incisions, which eventually gets incorporated into the surrounding skin or sloughs off after birth.
The fetus is then returned completely back into the uterus. The uterus is then closed in layers, followed by abdominal fascia and skin closure. The patient recovers in the obstetric postsurgical unit and is eventually discharged. A cesarean section delivery is scheduled for 37 weeks of gestational age.
As spina bifida is the only nonlethal disease for which fetal intervention is offered, the use of endoscopy has enabled mothers to return to vaginal delivery for the subsequent child with a decreased risk of uterine rupture or fetal demise. The initial fetoscopic work had challenging outcomes and there were issues with wound healing, resulting in returns to the operating room after delivery as well as fetal demise. This improved over time with experience.[34] There are limitations of the endoscopic technique, specifically the lack of instrument wrist articulation and dexterity at the point of interface with the patient. There was a high rate of amniotic fluid leakage and prematurity, and the neurologic outcomes were not reported, making it difficult to analyze the results fully.[35-37]
MATERNAL CONSIDERATIONS
The mother has to undergo two major operative procedures in a relatively short period of time with the associated perioperative risks. Only 1% required maternal transfusion at the time of open fetal surgery at Children’s Hospital of Philadelphia (CHOP).[38] The need for transfusion at the time of delivery ranged from 3.4% in the CHOP series to 9% in the MOMS trial[38,39] which are higher than routine cesarean deliveries. The thick, active portion of the fundal region is where fetal surgery is performed, so the risk of dehiscence or rupture was reported only in ∼1%.[39] Either of these scenarios could result in a catastrophic outcome for the mother and fetus. Therefore, long-term maternal reproductive planning must also be considered when determining candidacy for in utero repair.
LANDMARK OUTCOMES
Hydrocephalus
The most important primary outcome of fetal surgery and the MOMS trial was the need for shunt placement by 1 year of age. There was a slight discrepancy in the rates of shunting, due to independent surgeon discretion. There was a significant decrease in the rate of shunt placement in the prenatal group compared to the postnatal group, 40% versus 82%, respectively [Table 4].[40,41]
Maternal outcomes |
|
78/78 (100%) 20/78 (26%) 5/78 (6%) 7/78 (9%) 8/76 (11%) |
Fetal outcomes (primary) |
|
77/78 (99%) 76/77 (99%) 34.1±3.1 2383±688 28/77 (36%) |
Fetal outcomes (secondary) |
|
26/62 (42%) 18/62 (29%) 20/62 (32%) 45/70 (64%) 31 (40%) |
MOMS: Management of myelomeningocele study
The first 100 patients at CHOP showed that only 2.4% required an early shunt before discharge from neonatal intensive care unit.[38] This makes it a unique factor in prenatal patients as a delay in shunt placement not only reduces complications but also opens doors to alternative treatments like endoscopic third ventriculostomy at an older age.[42] This number goes up to 34% at 1 year of age (unpublished data).
A correlation between ventricular size and the requirement for shunts was also noted. Those prenatal patients with an atrial diameter of <10 mm had a shunt rate of 20%. If the ventricles were 10–15 mm, shunt rate was 45% and if >15 mm, shunt rate was 79%. This correlation was not noted in the post-natal group.
Chiari reversal
Hindbrain herniation is postulated to obstruct the 4th ventricular outflow, resulting in hydrocephalus. The compressive effects on the brain stem often lead to various respiratory, cardiovascular and feeding hindrances, which are also life-threatening.[43] About 75% of patients treated after the MOMS trial at CHOP had no herniation with 84% having mild or no herniation. In striking contrast, the postnatal group only 4% had no hind brain herniation and 33% had either mild or no herniation [Table 4].[38]
Motor status
Around 32% of prenatally treated patients were 2 levels better compared to only 12% of the postnatal group, in the MOMS trial. In the CHOP series, 30% prenatally operated babies had 2 level or better improvement.[44]
The MOMS trial also showed children in the prenatal group walked without support or assistive devices and had a higher mean functional rehabilitation evaluation of sensori-neurological outcomes (29% vs. 11%). Those with low lumbar and sacral lesions, less impairment in lower-limb function may be predicted. In fact, 43% of prenatally treated children were 1 level or better compared to the postnatal group [Table 4].[44]
Urological status
This was one outcome that unfortunately showed no significant improvement. Even though it did not reduce the need for clean intermittent catheterization (CIC), it was associated with significantly lesser vesicoureteral reflux, open bladder neck, bladder trabeculation, and ureteral dilation with significantly better bladder shape. A longer follow-up of this population which is ongoing in the MOMS-2 and 3 can determine efficacy of prenatal surgery and need for CIC and for urinary continence [Table 4].[45]
Functional and developmental status
Parents of prenatally operated babies report better adaptive behavior and higher quality of life Z-score. They also reported reduced negative mean family impact scores and better gross and fine motor functioning.[46] However, all children regardless of the type of surgery (pre or postnatal) are at risk for significant learning, motor, and functional challenges.
COMPLICATIONS
Fetal
Prematurity
The prenatal group had an average gestational age of delivery of 34.1 weeks versus 37.3 weeks in the postnatal group.[39] Even though the prenatal group had a higher rate of prematurity, it did not affect their neurodevelopmental outcomes.[47]
Tethering
The general underlying cause of tethered cord syndrome (TCS) secondary to MMC is that scar tissue formation at the repair site fixes the spinal cord in place, prohibiting its ascent with growth as in TCS from other causes.[48] This has led to multiple international guidelines[49,50] and the authors evaluating different closure techniques to reduce scar/adhesion formation and theoretically reduce TCS rates [Figure 4a-c].
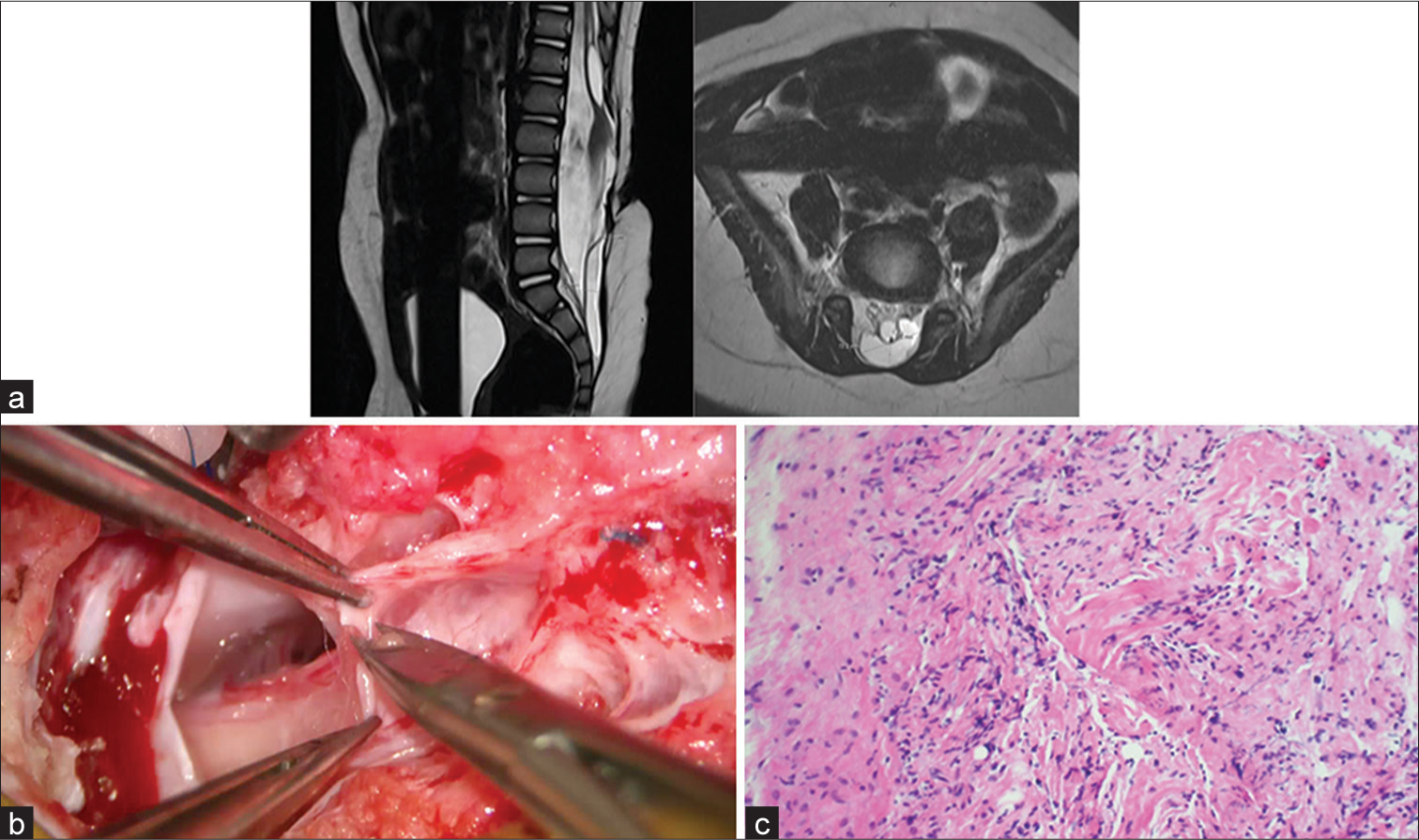
- Tethered cord syndrome (a) magnetic resonance imaging of the patulous spinal canal with conus medullaris extending beyond L3 and adherent to neural placode in addition to surrounding nerve roots. Associated syringohydromyelia with fatty filum within the sacral dural sleeve is also noted. (b) Intraoperative view of scar tissue during detethering surgery. (c) Shows the histopathological view of the resected scar tissue with fibrous tissue and inflammation during detethering surgery (Magnified 200×).
Inclusion cysts
Several articles report formation of inclusion cysts at the closure site following prenatal MMC closure.[51,52] While they also occur in those with postnatal closure done, they are usually asymptomatic. The resection of these lesions is technically more difficult due to their variations and unique anatomy [Figure 5a-c].
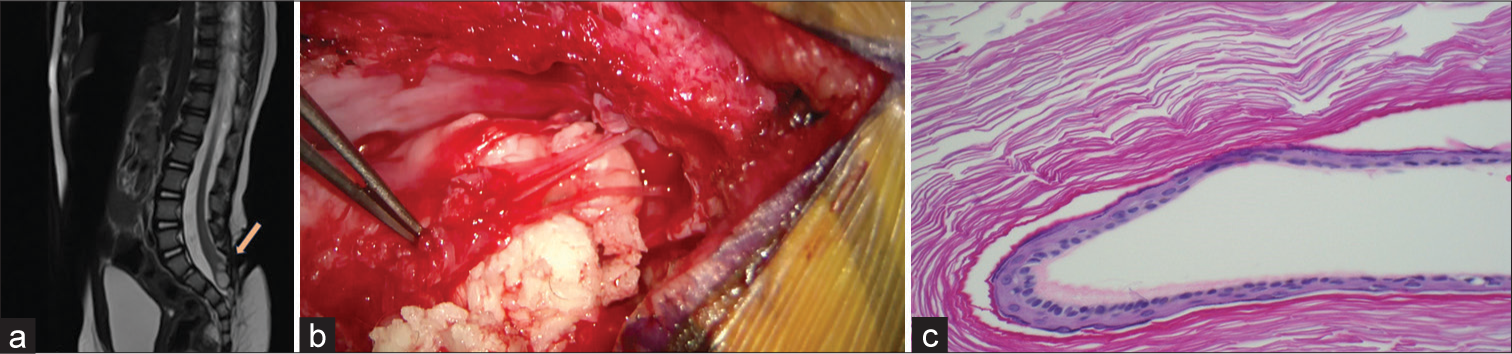
- Spinal cord inclusion cyst (a) T2-weighted sagittal image of lumbosacral spine showing mixed intensity distal lesion within the spinal cord, suggestive of inclusion cyst (orange arrow). (b) Intraoperative photo of epidermoid cyst during resection/detethering. (c) An Epidermoid histopathology mount (magnified 100×) showing a layer of keratinized squamous epithelial lining the cyst cavity containing a granular cell layer.
Maternal
A significant increase in placental abruption, pulmonary edema, and blood transfusion was noted in the prenatal repair group. Despite meticulous multilayered closure, the expected oligohydramnios and varying degree of uterine dehiscence were seen in 20% and 11%, respectively. Chorioamniotic membrane separation, which increases the risk of spontaneous rupture of membranes, was observed in one-third of pregnancies that underwent prenatal surgery. However, 65% of the prenatal group had an intact, well-healed hysterotomy at the time of C-section.
Soni et al.[53] demonstrated a linear link of gestational age at prenatal surgery to the incidence of chorioamniotic membrane separation (CMS) and preterm premature rupture of membranes (PPROM) with a risk of 60% at 20–21 weeks and this drastically reduces to 0% at 25 + 6 weeks, thus suggesting delaying fetal repair until 23 weeks not only decrease complications but also decrease preterm deliveries. However, despite these complications, there were no maternal mortalities.
Recent advances in minimally invasive fetoscopy may help decrease the incidence of preterm premature rupture of membranes (PPROM) and preterm deliveries, thus improving maternal morbidity and outcomes.
POSTFETAL REPAIR PROTOCOLS
It is important to provide the expecting parents a comprehensive guide of their unborn child encompassing all aspects, from fetal to adult life. As discussed earlier, though fetal surgery improves the long-term quality of life compared to postnatal surgery, these benefits are also accompanied by their shortcomings.
The mother is counseled regarding the two uterine scars (one from the hysterotomy during fetal repair, and one from the delivery). This also puts her at a risk of abnormal placentation, uterine rupture, and uterine dehiscence which may even affect further pregnancies.[54,55]
The enhanced recovery after surgery (ERAS) protocols are evidence-based care plans to ensure speedy patient recovery and shorter hospitalization. A questionnaire was completed by 46 centers in 17 countries performing open and fetoscopic surgery.[56]
The mother is admitted under intensive care unit care for 24–48 h, followed by the antenatal ward for typically 5–7 days. The ERAS emphasized the importance of the use of regional analgesia (epidural or transversus abdominis plane block) with good post-operative intravenous or oral analgesic cover. Pre-emptive prophylaxis with antibiotics and post-operative thrombosis prophylaxis with low-molecular-weight heparin and ted stockings are initiated with early mobilization. The catheter is usually removed on post-operative day 1, and early feeding on the day of the surgery itself is encouraged. Tocolysis is continued and incentivized throughout the pregnancy. The mother is also advised to limit strenuous activities.
During her hospital stay, fetal ultrasounds are done everyday till discharge to monitor the viable fetus, its movements, and the operative site.[57]
ON DISCHARGE
Post-discharge, the neonatal and pediatric team is kept informed at all times. Cranial USG and OFC are evaluated daily till discharge and after every fortnight from discharge. A 1–2 weekly USG and review is performed for liquor volume, Dopplers, and cervical length. Furthermore, a 4 weekly USG review for liquor volume, Dopplers, cervical length, and fetal neurological growth is done. A fetal MRI is advised within 30–34 weeks of gestation. The elective caesarian is performed by 36–40 weeks with fetal steroid coverage.
In the event of a preterm labor at any point following the fetal surgery, an emergency cesarean is done, due to the increased risk of uterine rupture with recent hysterotomy. The hysterotomy site is inspected and resutured if dehiscent. Following fetal surgery for spina bifida, an interval of 2 years is suggested for all future deliveries.[57]
CONCLUSION
At present, fetal surgery for the repair of spina bifida is not done in India. Prenatal surgery is an effective management strategy for carefully selected patients in an experienced center. Not only do the prenatal babies have a lower mortality rate but also improved motor function with the likelihood of independent walking. Reducing the need for CSF diversion is due to the reduction in hindbrain herniation rates. Even though intrauterine surgery has now achieved its “quarter-century” journey, one must carefully weigh the potential benefits and balance them against the risks of prematurity and maternal morbidity. The ideal goal should be to eliminate hydrocephalus, normalize neurological function, term delivery, and the ability of the mother to return to normal risk of subsequent pregnancy.
As the benefits of fetal surgery have become more widely accepted, we propagate that given the prevalence of spina bifida, especially in India, the assembly of a fetal team is both a resource and expertise intensive investment, as it requires the superlative focus of all involved specialties. It must be at the forefront of any institution to offer fetal surgery.
Ethical approval
Institutional Review Board approval is not required.
Declaration of patient consent
Patient’s consent not required as patients identity is not disclosed or compromised.
Conflicts of interest
There are no conflicts of interest.
Use of artificial intelligence (AI)-assisted technology for manuscript preparation
The authors confirm that there was no use of artificial intelligence (AI)-assisted technology for assisting in the writing or editing of the manuscript and no images were manipulated using AI.
Financial support and sponsorship
Nil.
References
- Fetal surgery for spina bifida aperta. Arch Dis Child Fetal Neonatal Ed. 2018;103:F589-95.
- [CrossRef] [PubMed] [Google Scholar]
- Decline in the prevalence of spina bifida and anencephaly by race/ethnicity: 1995-2002. Pediatrics. 2005;116:580-6.
- [CrossRef] [PubMed] [Google Scholar]
- Global hydrocephalus epidemiology and incidence: Systematic review and meta-analysis. J Neurosurg. 2019;130:1065-79.
- [CrossRef] [PubMed] [Google Scholar]
- Congenital malformations in India In: Satyavati GV, ed. Peoples of India. Some genetical aspects. New Delhi: Indian Council of Medical Research; 1983. p. :70.
- [Google Scholar]
- Systematic review of birth prevalence of neural tube defects in India. Birth Defects Res A Clin Mol Teratol. 2013;97:437-43.
- [CrossRef] [PubMed] [Google Scholar]
- Prevalence of neural tube defects in a rural area of north India from 2001 to 2014: A population-based survey. Birth Defects Res. 2017;109:203-10.
- [CrossRef] [PubMed] [Google Scholar]
- Incidence of neural tube defects of Lucknow over a 10 year period from 1982-1991. Indian J Med Res. 1994;99:223-6.
- [Google Scholar]
- Neural tube defect: Epidemiologic and demographic implication. IOSR J Dent Med Sci. 2014;13:1-4.
- [CrossRef] [Google Scholar]
- Prevention of neural tube defects: Results of the medical research council Vitamin Study. MRC Vitamin study research group. Lancet. 1991;338:131-7.
- [CrossRef] [Google Scholar]
- Fetal spina bifida in a mouse model: Loss of neural function in utero. J Neurosurg. 2007;106:213-21.
- [CrossRef] [PubMed] [Google Scholar]
- The paralysis associated with myelomeningocele: Clinical and experimental data implicating a preventable spinal cord injury. Neurosurgery. 1990;26:987-92.
- [CrossRef] [PubMed] [Google Scholar]
- Shunt complications in the first postoperative year in children with meningomyelocele. Childs Nerv Syst. 1996;12:748-54.
- [CrossRef] [PubMed] [Google Scholar]
- Intrauterine treatment of spina bifida: Primate model. Z Kinderchir. 1984;39:259-61.
- [CrossRef] [PubMed] [Google Scholar]
- In utero surgery rescues neurological function at birth in sheep with spina bifida. Nat Med. 1995;1:342-7.
- [CrossRef] [PubMed] [Google Scholar]
- Correction of hindbrain herniation and anatomy of the vermis after in utero repair of myelomeningocele in sheep. J Pediatr Surg. 2003;38:451-8.
- [CrossRef] [PubMed] [Google Scholar]
- Hindbrain herniation develops in surgically created myelomeningocele but is absent after repair in fetal lambs. Am J Obstet Gynecol. 2000;183:1119-23.
- [CrossRef] [PubMed] [Google Scholar]
- Early fetal leg movements in myelomeningocele. Lancet. 1986;1:917-8.
- [CrossRef] [PubMed] [Google Scholar]
- Perinatal motor behaviour and neurological outcome in spina bifida aperta. Early Hum Dev. 1997;50:27-37.
- [CrossRef] [PubMed] [Google Scholar]
- Creation of myelomeningocele in utero: A model of functional damage from spinal cord exposure in fetal sheep. J Pediatr Surg. 1995;30:1028-32.
- [CrossRef] [PubMed] [Google Scholar]
- The university of California at San Francisco fetal treatment center: A personal perspective. Fetal Diagn Ther. 2004;19:513-24.
- [CrossRef] [PubMed] [Google Scholar]
- In utero allogeneic bone transplantation in primates: Roentgenographic and histological observations. Transplantation. 1981;32:96-100.
- [CrossRef] [PubMed] [Google Scholar]
- The fetal neural tube: Is intervention progress? Z Kinderchir. 1985;40(Suppl 1):53-7.
- [CrossRef] [PubMed] [Google Scholar]
- The spinal cord lesion in human fetuses with myelomeningocele: Implications for fetal surgery. J Pediatr Surg. 1997;32:448-52.
- [CrossRef] [PubMed] [Google Scholar]
- Update on prenatal diagnosis and fetal surgery for myelomeningocele. Arch Argent Pediatr. 2021;119:e215-28.
- [CrossRef] [Google Scholar]
- Assessment of intracranial translucency (IT) in the detection of spina bifida at the 11-13 week scan. Ultrasound Obstet Gynecol. 2009;34:249-52.
- [CrossRef] [PubMed] [Google Scholar]
- Sonographic detection of central nervous system defects in the first trimester of pregnancy: First-trimester detection of CNS malformations. Prenat Diagn. 2016;36:266-73.
- [CrossRef] [PubMed] [Google Scholar]
- Crash sign: New first-trimester sonographic marker of spina bifida. Ultrasound Obstet Gynecol. 2019;54:740-5.
- [CrossRef] [PubMed] [Google Scholar]
- Prenatal diagnosis of terminal myelocystocele in the fetal surgery era: Case report. Neurosurgery. 2002;50:1152-4. discussion 1154-5
- [CrossRef] [PubMed] [Google Scholar]
- Prenatal diagnosis and postnatal outcome of fetal spinal defects without Arnold-Chiari II malformation. Prenat Diagn. 2009;29:1050-7.
- [CrossRef] [PubMed] [Google Scholar]
- Fetal MRI in the evaluation of intrauterine myelomeningocele. Pediatr Neurosurg. 2000;32:124-31.
- [CrossRef] [PubMed] [Google Scholar]
- Fetal spine findings on MRI and associated outcomes in children with open neural tube defects. AJR Am J Roentgenol. 2011;197:W956-61.
- [CrossRef] [PubMed] [Google Scholar]
- Endoscopic coverage of fetal myelomeningocele in utero. Am J Obstet Gynecol. 1999;180:153-8.
- [CrossRef] [PubMed] [Google Scholar]
- Fetal endoscopic myelomeningocele closure preserves segmental neurological function. Dev Med Child Neurol. 2012;54:15-22.
- [CrossRef] [PubMed] [Google Scholar]
- Percutaneous minimal-access fetoscopic surgery for spina bifida aperta. Part II: Maternal management and outcome. Ultrasound Obstet Gynecol. 2014;44:525-31.
- [CrossRef] [PubMed] [Google Scholar]
- A randomized trial of prenatal versus postnatal repair of myelomeningocele. N Engl J Med. 2011;364:993-1004.
- [CrossRef] [PubMed] [Google Scholar]
- Prenatal surgery for myelomeningocele and the need for cerebrospinal fluid shunt placement. J Neurosurg Pediatr. 2015;16:613-20.
- [CrossRef] [PubMed] [Google Scholar]
- Fetoscopic coverage of experimental myelomeningocele in sheep using a patch with surgical sealant. Eur J Obstet Gynecol Reprod Biol. 2011;156:171-6.
- [CrossRef] [PubMed] [Google Scholar]
- Endoscopic third ventriculostomy in the treatment of childhood hydrocephalus in Uganda: Report of a scoring system that predicts success. J Neurosurg Pediatr. 2010;5:143-8.
- [CrossRef] [PubMed] [Google Scholar]
- The cause of Chiari II malformation: A unified theory. Pediatr Neurosci. 1989;15:1-12.
- [CrossRef] [PubMed] [Google Scholar]
- Fetal myelomeningocele repair: The post-MOMS experience at the Children's Hospital of Philadelphia. Fetal Diagn Ther. 2015;37:235-40.
- [CrossRef] [PubMed] [Google Scholar]
- Bladder function after fetal surgery for myelomeningocele. Pediatrics. 2015;136:e906-13.
- [CrossRef] [PubMed] [Google Scholar]
- Prenatal repair of myelomeningocele and school-age functional outcomes. Pediatrics. 2020;145:e20191544.
- [CrossRef] [PubMed] [Google Scholar]
- Long-term neurofunctional outcome, executive functioning, and behavioral adaptive skills following fetal myelomeningocele surgery. Am J Obstet Gynecol. 2016;214:269.e1-8.
- [CrossRef] [PubMed] [Google Scholar]
- Tethered Cord syndrome after myelomeningocele repair: A literature update. Cureus. 2020;12:e10949.
- [CrossRef] [Google Scholar]
- Towards guideline-based management of tethered cord syndrome in spina bifida: A global health paradigm shift in the era of prenatal surgery. Neurospine. 2019;16:715-27.
- [CrossRef] [PubMed] [Google Scholar]
- Intradural inclusion cysts following in utero closure of myelomeningocele: Clinical implications and follow-up findings. J Neurosurg Pediatr. 2008;2:406-13.
- [CrossRef] [PubMed] [Google Scholar]
- Dermoid inclusion cysts and early spinal cord tethering after fetal surgery for myelomeningocele. N Engl J Med. 2002;347:256-9.
- [CrossRef] [PubMed] [Google Scholar]
- Chorioamniotic membrane separation and preterm premature rupture of membranes complicating in utero myelomeningocele repair. Am J Obstet Gynecol. 2016;214:7.e1-7.
- [CrossRef] [PubMed] [Google Scholar]
- Reproductive outcomes in subsequent pregnancies after a pregnancy complicated by open maternal-fetal surgery (1996-2007) Am J Obstet Gynecol. 2010;203:209.e1-6.
- [CrossRef] [PubMed] [Google Scholar]
- Subsequent pregnancy outcomes after open maternal-fetal surgery for myelomeningocele. Am J Obstet Gynecol. 2019;220:494.e1-7.
- [CrossRef] [PubMed] [Google Scholar]
- Enhanced recovery after surgery (ERAS) in gynecologic oncology: An international survey of peri-operative practice. Int J Gynecol Cancer. 2020;30:1471-8.
- [CrossRef] [PubMed] [Google Scholar]
- Fetal surgery for open spina bifida. Obstet Gynaecol. 2019;21:271-82.
- [CrossRef] [PubMed] [Google Scholar]